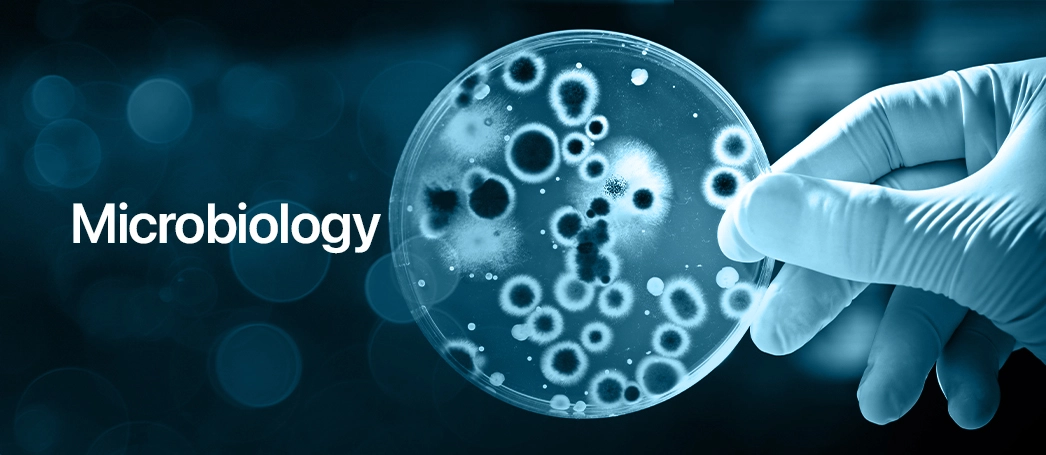
Microbiology: Foundations and Applications in Bioinformatics
March 31, 2024Introduction to Microbiology
Scope of Microbiology
Microbiology is a vast field that encompasses the study of microscopic organisms, including bacteria, viruses, fungi, protozoa, and algae. It has numerous branches and applications, including:
- Medical Microbiology: Study of microorganisms that cause infectious diseases in humans, including bacteria, viruses, fungi, and parasites. It involves the diagnosis, treatment, and prevention of infectious diseases.
- Environmental Microbiology: Study of the roles of microorganisms in the environment, including their roles in nutrient cycling, biodegradation, and environmental impact.
- Industrial Microbiology: Application of microorganisms in industrial processes, such as fermentation, waste treatment, bioremediation, and the production of food, beverages, antibiotics, and enzymes.
- Agricultural Microbiology: Study of microorganisms in soil fertility, plant nutrition, and plant diseases, with applications in agriculture, horticulture, and forestry.
- Food Microbiology: Study of microorganisms in food production, processing, preservation, and safety, including the prevention of foodborne illnesses.
- Microbial Genetics: Study of the genetics of microorganisms, including gene expression, genetic transfer, and genetic engineering for biotechnological applications.
- Immunology: Study of the immune system, including how it responds to infectious agents and how it can be manipulated for the treatment of diseases.
- Microbial Ecology: Study of the interactions between microorganisms and their environments, including their roles in ecosystems, biogeochemical cycles, and climate change.
- Veterinary Microbiology: Study of microorganisms that affect animal health, including those that cause diseases in livestock and pets.
- Water Microbiology: Study of microorganisms in water, including their roles in water quality, purification, and wastewater treatment.
Microbiology plays a crucial role in various fields, including medicine, agriculture, food production, biotechnology, environmental science, and veterinary science. Its scope continues to expand with advancements in technology and our understanding of microbial life.
History of Microbiology
The history of microbiology dates back to ancient times when people first observed microorganisms without understanding their nature. However, modern microbiology began to emerge in the 17th century with the advent of the microscope and the work of pioneering scientists. Here are some key milestones in the history of microbiology:
- Antonie van Leeuwenhoek (1632-1723): A Dutch scientist who is often considered the father of microbiology. He was the first to observe and describe microorganisms, which he called “animalcules,” using a simple microscope of his own design.
- Francesco Redi (1626-1697): An Italian physician who conducted experiments to refute the theory of spontaneous generation, which posited that living organisms could arise spontaneously from non-living matter. His work laid the foundation for the field of microbiology.
- Louis Pasteur (1822-1895): A French chemist and microbiologist who made several groundbreaking discoveries in the field of microbiology. He is known for his experiments that disproved the theory of spontaneous generation, his development of the process of pasteurization to prevent food spoilage, and his development of vaccines for rabies and anthrax.
- Robert Koch (1843-1910): A German physician and microbiologist who is considered one of the founders of modern bacteriology. He developed a series of postulates, known as Koch’s postulates, to establish the causal relationship between a microorganism and a disease. He also identified the causative agents of several diseases, including anthrax, tuberculosis, and cholera.
- Joseph Lister (1827-1912): A British surgeon who is known for pioneering antiseptic surgery. He introduced the use of carbolic acid (phenol) as an antiseptic to sterilize surgical instruments and clean wounds, reducing the risk of infection.
- Martinus Beijerinck (1851-1931): A Dutch microbiologist who discovered the first virus, tobacco mosaic virus, and coined the term “virus” to describe it. He also made significant contributions to the study of nitrogen fixation and soil microbiology.
- Sergei Winogradsky (1856-1953): A Russian microbiologist who is known for his work in environmental microbiology and microbial ecology. He discovered several new species of bacteria and pioneered the study of chemolithotrophy, the process by which microorganisms obtain energy from inorganic compounds.
These pioneering scientists laid the foundation for the field of microbiology, which has since grown to encompass a wide range of disciplines and applications. Today, microbiology plays a crucial role in medicine, agriculture, food production, biotechnology, environmental science, and many other fields.
Spontaneous generation
Spontaneous generation was a widely held belief that living organisms could arise spontaneously from non-living matter. This idea was prevalent in ancient times and was supported by observations such as the appearance of maggots on decaying meat or the sudden appearance of fish in dried-up ponds after rain.
The theory of spontaneous generation was challenged and eventually disproved through a series of experiments by scientists such as Francesco Redi and Louis Pasteur. Redi conducted experiments in the 17th century showing that maggots only appeared on meat when flies were allowed to land on it, refuting the idea that they arose spontaneously from the meat.
Pasteur’s experiments in the 19th century provided further evidence against spontaneous generation. He demonstrated that microorganisms did not spontaneously generate in nutrient broths when exposed to air, but rather were carried in the air and could be prevented from entering the broth by proper sterilization techniques. This led to the development of aseptic techniques and the understanding that microorganisms come from pre-existing microorganisms.
The disproval of spontaneous generation was a significant milestone in the history of microbiology, as it laid the foundation for the modern understanding of the microbial world and the development of microbiological techniques such as sterilization, pasteurization, and aseptic technique.
Germ theory of diseases
The germ theory of disease is the concept that microorganisms, primarily bacteria and viruses, are the cause of many diseases. This theory revolutionized medicine and our understanding of infectious diseases. Here’s an overview of its development and impact:
- Antonie van Leeuwenhoek (1632-1723): While Leeuwenhoek’s work predates the formal germ theory, his observations of microorganisms laid the groundwork for understanding their role in disease.
- Louis Pasteur (1822-1895): Pasteur’s experiments in the mid-19th century provided strong evidence for the germ theory. He demonstrated that microorganisms are present in the air and can contaminate sterile solutions, leading to spoilage. He also showed that heating liquids to specific temperatures could kill these microorganisms, a process known as pasteurization.
- Robert Koch (1843-1910): Koch’s work further solidified the germ theory and provided a framework for identifying the specific microorganisms responsible for particular diseases. He developed a series of postulates (Koch’s postulates) that are used to establish a causal relationship between a microorganism and a disease.
- Impact on Medicine: The acceptance of the germ theory revolutionized medicine by providing a scientific basis for understanding and treating infectious diseases. It led to improvements in sanitation, hygiene, and medical practices, such as sterilization and aseptic techniques.
- Vaccination: The germ theory also contributed to the development of vaccines. By understanding that microorganisms cause diseases, scientists were able to develop vaccines to prevent infections by stimulating the immune system to recognize and attack specific pathogens.
- Antibiotics: The germ theory paved the way for the development of antibiotics, which are substances that can kill or inhibit the growth of bacteria. Antibiotics have been instrumental in treating bacterial infections and saving countless lives.
Overall, the germ theory of disease has had a profound impact on public health and medicine, leading to significant advancements in our ability to prevent, diagnose, and treat infectious diseases.
Cell theory
Cell theory is a fundamental theory in biology that states:
- All living organisms are composed of one or more cells.
- The cell is the basic unit of structure and organization in organisms.
- Cells arise from pre-existing cells through cell division.
The cell theory was proposed in the 19th century and is considered one of the foundations of modern biology. Here’s a brief overview of its development:
- Robert Hooke (1635-1703): In 1665, Robert Hooke, an English scientist, discovered cells while examining a thin slice of cork under a microscope. He coined the term “cell” to describe the tiny compartments he observed, which reminded him of the cells inhabited by monks in a monastery.
- Antonie van Leeuwenhoek (1632-1723): A Dutch scientist, Leeuwenhoek made significant improvements to the microscope and was the first to observe and describe single-celled organisms, which he called “animalcules.”
- Matthias Schleiden (1804-1881) and Theodor Schwann (1810-1882): In the 1830s, Schleiden, a German botanist, and Schwann, a German zoologist, independently formulated the cell theory based on their observations and research. Schleiden concluded that all plant tissues are composed of cells, while Schwann proposed that animals are also made up of cells.
- Rudolf Virchow (1821-1902): A German physician, Virchow, later added to the cell theory by stating that cells can only arise from pre-existing cells, challenging the idea of spontaneous generation.
The cell theory laid the groundwork for the field of cell biology, which studies the structure, function, and behavior of cells. It has since been expanded and refined with advancements in technology, leading to a deeper understanding of cellular processes and the development of modern biotechnology and medicine.
Contributions
Antonie van Leeuwenhoek (1632-1723) was a Dutch scientist and tradesman who made pioneering contributions to the field of microscopy and microbiology. His discoveries laid the foundation for the field of microbiology. Some of his key contributions include:
- Microscopic Observations: Leeuwenhoek was the first to observe and describe microorganisms, which he called “animalcules.” Using microscopes of his own design, he observed bacteria, protozoa, and other microorganisms for the first time, opening up a new world of the unseen.
- Advancements in Microscopy: Leeuwenhoek made significant improvements to the design and construction of microscopes, achieving magnifications of up to 270 times, far surpassing the capabilities of other microscopes of his time.
- Discovery of Sperm Cells: Leeuwenhoek was the first to observe and describe sperm cells, which he called “animalcules of the semen,” in human semen and other animals.
- Observation of Blood Cells: He was also the first to observe and describe red blood cells, which he called “red globules,” in various animals.
- Discovery of Microscopic Structures: Leeuwenhoek’s observations extended beyond microorganisms. He also described and illustrated microscopic structures in a variety of materials, including plants, insects, and minerals.
- Scientific Communication: Despite his lack of formal scientific training, Leeuwenhoek communicated his findings to the scientific community through letters to the Royal Society of London, where they were published and widely disseminated, contributing to the advancement of scientific knowledge.
Antonie van Leeuwenhoek’s contributions to microscopy and microbiology were groundbreaking and laid the foundation for the development of these fields. His meticulous observations and innovative approach to microscopy opened up new avenues of scientific inquiry and revolutionized our understanding of the microscopic world.
Here is a brief overview of the contributions of each of these scientists:
- Joseph Lister (1827-1912): Known as the father of antiseptic surgery, Lister pioneered the use of antiseptic agents to prevent surgical infections. His work significantly reduced post-operative infections and mortality rates.
- Robert Koch (1843-1910): A German physician and microbiologist, Koch is known for his work on identifying the causative agents of anthrax, tuberculosis, and cholera. He developed Koch’s postulates, a series of criteria to establish the causal relationship between a microorganism and a disease.
- Louis Pasteur (1822-1895): A French chemist and microbiologist, Pasteur made significant contributions to the fields of microbiology and immunology. He developed the process of pasteurization to prevent food spoilage and the spread of disease. He also developed vaccines for rabies and anthrax.
- Edward Jenner (1749-1823): An English physician, Jenner is known for developing the smallpox vaccine. His work laid the foundation for the concept of vaccination and the eventual eradication of smallpox.
- John Tyndall (1820-1893): An Irish physicist, Tyndall made important contributions to our understanding of the properties of air and the transmission of light through different substances. He also conducted experiments that demonstrated the presence of heat-resistant microbial spores in the air.
- Sergei N. Winogradsky (1856-1953): A Russian microbiologist, Winogradsky is known for his work in environmental microbiology and microbial ecology. He discovered several new species of bacteria and pioneered the study of chemolithotrophy.
- Alexander Fleming (1881-1955): A Scottish biologist and pharmacologist, Fleming discovered the antibiotic properties of penicillin. His discovery revolutionized the treatment of bacterial infections and led to the development of many other antibiotics.
Each of these scientists made significant contributions to the fields of microbiology, immunology, and medicine, advancing our understanding of infectious diseases and improving public health.
Microbial cell structure and function
Cell Size, Arrangement, and Shape:
- Bacterial cells are typically much smaller than eukaryotic cells, ranging from about 0.2 to 2.0 micrometers in diameter.
- Bacteria can be found in various arrangements, including cocci (spherical), bacilli (rod-shaped), and spirilla (spiral-shaped).
- The shape of bacterial cells can vary greatly and is often influenced by the cell wall and cytoskeleton.
Capsule, Slime, and Pili:
- Capsules are protective layers of polysaccharides or proteins that surround some bacterial cells, providing protection against host immune responses.
- Slime layers are similar to capsules but are more loosely organized and are involved in attachment to surfaces.
- Pili (or fimbriae) are hair-like appendages that extend from the surface of some bacterial cells and are involved in attachment to surfaces or other cells.
Spores:
- Spores are specialized, dormant structures formed by some bacteria in response to unfavorable environmental conditions.
- Spores are highly resistant to heat, desiccation, and chemical agents, allowing the bacteria to survive harsh conditions.
- Examples of spore-forming bacteria include Bacillus and Clostridium species.
Gram-Negative & Gram-Positive Cell Wall and Membrane:
- Gram-negative bacteria have a thin peptidoglycan layer in their cell wall and an outer membrane composed of lipopolysaccharides (LPS).
- Gram-positive bacteria have a thick peptidoglycan layer in their cell wall but lack an outer membrane.
- The Gram stain is a common laboratory technique used to differentiate between Gram-negative and Gram-positive bacteria based on differences in cell wall structure.
Periplasmic Space:
- The periplasmic space is a region between the inner and outer membranes of Gram-negative bacteria.
- It contains a variety of proteins and enzymes involved in nutrient uptake, cell wall synthesis, and detoxification.
Viruses:
- Viruses are infectious agents that are much smaller than bacteria and consist of genetic material (DNA or RNA) surrounded by a protein coat (capsid).
- Viruses are obligate intracellular parasites, meaning they can only replicate inside host cells.
- They infect a wide range of organisms, including animals, plants, fungi, and bacteria.
Mycoplasma:
- Mycoplasma are bacteria that lack a cell wall, making them resistant to antibiotics that target cell wall synthesis.
- They are among the smallest known free-living organisms and have a simple cell structure.
- Mycoplasma species can cause diseases such as pneumonia and urinary tract infections in humans and animals.
Eukaryotic Microbes:
- Eukaryotic microbes include protists, fungi, and algae, which are single-celled organisms with a complex internal structure.
- Protists are a diverse group of eukaryotes that include both photosynthetic and non-photosynthetic organisms.
- Fungi are a group of eukaryotes that include yeasts, molds, and mushrooms. They play important roles in decomposition, nutrient cycling, and food production.
- Algae are photosynthetic eukaryotes that are important primary producers in aquatic ecosystems and also play a role in carbon fixation and oxygen production.
Microbial Taxonomy
Taxonomy: principle and its types
Taxonomy is the science of classifying and naming organisms based on their similarities and evolutionary relationships. The principle of taxonomy is to organize the diversity of life into hierarchical categories that reflect evolutionary history. The primary goal of taxonomy is to provide a system of classification that is logical, easily understood, and universally applicable.
There are several types of taxonomy, including:
- Hierarchical Taxonomy: This type of taxonomy organizes organisms into a hierarchical structure based on their characteristics. The highest level is the domain, followed by kingdom, phylum, class, order, family, genus, and species.
- Phenetic Taxonomy: Phenetic taxonomy classifies organisms based on their overall similarity in physical and biochemical characteristics. This approach does not consider evolutionary relationships and relies on observable traits.
- Cladistic Taxonomy: Cladistic taxonomy, also known as phylogenetic taxonomy, classifies organisms based on their evolutionary relationships. It uses cladograms, or branching diagrams, to show the relationship between different groups of organisms based on shared derived characteristics.
- Numerical Taxonomy: Numerical taxonomy uses statistical methods to analyze large sets of data on characteristics of organisms to classify them. This approach is based on quantitative data and does not rely on subjective assessments of similarity.
- Alpha Taxonomy: Alpha taxonomy is concerned with the identification and classification of individual species. It involves describing new species and assigning them to existing taxonomic groups based on their similarities and differences.
- Beta Taxonomy: Beta taxonomy deals with the broader classification of organisms beyond the species level. It focuses on the relationships between different species and higher taxonomic groups.
- Polyphasic Taxonomy: Polyphasic taxonomy integrates multiple sources of data, including morphology, genetics, ecology, and biochemistry, to classify organisms. This approach provides a more comprehensive understanding of evolutionary relationships.
Taxonomy plays a crucial role in biology by providing a framework for understanding the diversity of life and its evolutionary history. It helps scientists organize and communicate information about organisms, study their relationships, and make predictions about their behavior and characteristics.
Classical approach
The classical approach to taxonomy includes several methods for classifying organisms based on different types of data. These methods include numerical, chemical, serological, and genetic approaches:
- Numerical Taxonomy: Numerical taxonomy uses statistical methods to analyze quantitative data on the characteristics of organisms. This approach groups organisms based on similarities in their measurable traits, such as morphology, physiology, or behavior. Cluster analysis and similarity coefficients are commonly used in numerical taxonomy to determine the degree of similarity between organisms and group them accordingly.
- Chemical Taxonomy: Chemical taxonomy involves analyzing the chemical composition of organisms to classify them. This can include studying the composition of cell walls, membranes, proteins, lipids, and other biochemical markers. Chemical taxonomy is particularly useful for identifying microorganisms and distinguishing between closely related species.
- Serological Taxonomy: Serological taxonomy uses serological techniques, such as immunodiffusion and enzyme-linked immunosorbent assay (ELISA), to study the antigenic properties of organisms. By analyzing the immune response of organisms to specific antigens, serological taxonomy can help classify organisms based on their antigenic similarities and differences.
- Genetic Taxonomy: Genetic taxonomy involves studying the genetic material of organisms, such as DNA or RNA, to classify them. This can include analyzing genetic sequences, such as those of specific genes or entire genomes, to determine evolutionary relationships between organisms. Genetic taxonomy is particularly useful for studying the evolutionary history of organisms and identifying genetic markers for classification.
These classical approaches to taxonomy have been widely used in the past and have provided valuable insights into the classification and identification of organisms. However, modern taxonomy increasingly relies on molecular techniques, such as DNA sequencing, for more precise and comprehensive classifications.
Bacterial taxonomy
Bergey’s Manual of Systematic Bacteriology is a renowned reference work in bacterial taxonomy. It provides comprehensive information on the classification, identification, and characterization of bacteria. The manual is organized into several volumes, with each volume focusing on different groups of bacteria.
- Volume 1: The Archaea and the Deeply Branching and Phototrophic Bacteria: This volume covers the Archaea domain and includes information on the deeply branching and phototrophic bacteria. It provides detailed descriptions of the taxonomy, morphology, physiology, and ecology of these groups.
- Volume 2: The Proteobacteria: This volume focuses on the Proteobacteria, which is a large and diverse group of bacteria that includes many medically, industrially, and ecologically important species. It provides detailed information on the classification, identification, and characterization of Proteobacteria.
- Volume 3: The Firmicutes: This volume covers the Firmicutes, a phylum of bacteria that includes many important human pathogens, such as Clostridium difficile and Staphylococcus aureus. It provides comprehensive information on the taxonomy, morphology, physiology, and ecology of Firmicutes.
- Volume 4: The Bacteroidetes, Spirochaetes, Tenericutes (Mollicutes), Acidobacteria, Fibrobacteres, Fusobacteria, Dictyoglomi, Gemmatimonadetes, Lentisphaerae, Verrucomicrobia, Chlamydiae, and Planctomycetes: This volume covers a diverse range of bacterial phyla, including the Bacteroidetes, Spirochaetes, Tenericutes, Acidobacteria, Fibrobacteres, Fusobacteria, Dictyoglomi, Gemmatimonadetes, Lentisphaerae, Verrucomicrobia, Chlamydiae, and Planctomycetes. It provides detailed descriptions of the taxonomy, morphology, physiology, and ecology of these groups.
Bergey’s Manual of Systematic Bacteriology is widely used by microbiologists, taxonomists, and researchers as a reference for the classification and identification of bacteria. It is considered a definitive resource in the field of bacterial taxonomy and is regularly updated to reflect advances in the field.
Methods and Techniques in Microbiology
Microscopy
Microscopy is the science of using microscopes to observe objects and details that are too small to be seen with the naked eye. There are several types of microscopes, each with its own principles and applications:
- Light Microscope: Also known as an optical microscope, this type of microscope uses visible light to illuminate and magnify samples. It is commonly used in biology, medicine, and materials science.
- Phase Contrast Microscope: This type of microscope enhances the contrast of transparent and colorless objects by exploiting differences in the phase of light passing through the sample.
- Dark Field Microscope: Dark field microscopy illuminates the sample with light that is scattered by the sample, rather than directly transmitted through it. This creates a bright image of the object against a dark background, making it useful for observing unstained samples.
- Bright Field Microscope: This is the most common type of microscope, where the specimen is illuminated with white light, and the image is viewed against a bright background. It is suitable for observing stained or naturally pigmented samples.
- Fluorescence Microscope: This type of microscope uses fluorescence to visualize structures within a specimen. Fluorescent molecules in the sample emit light of a different color when illuminated with specific wavelengths, allowing for the visualization of specific structures or molecules.
- Interference Microscope (Stereo Microscope): This type of microscope uses interference patterns to enhance the contrast and three-dimensional appearance of the sample. It is commonly used for dissecting and examining larger specimens at low magnifications.
- Confocal Microscope: Confocal microscopy uses a pinhole to eliminate out-of-focus light, allowing for the acquisition of sharp, high-contrast images of thick specimens. It is often used in biology and materials science for imaging thick specimens or for 3D imaging.
- Electron Microscope (TEM and SEM): Electron microscopes use a beam of electrons to illuminate the specimen, providing much higher magnification and resolution than light microscopes. Transmission Electron Microscopy (TEM) transmits electrons through a thin section of the specimen to create an image, while Scanning Electron Microscopy (SEM) scans a focused electron beam across the surface of the specimen to create a detailed 3D image.
Each type of microscope has its advantages and limitations, and the choice of microscope depends on the specific requirements of the sample and the desired level of detail and resolution.
Nutrition, Growth, and Culturing
Microbiological media
Microbiological media are nutrient-rich substances used to grow and cultivate microorganisms in the laboratory. These media provide the necessary nutrients, pH, and other conditions to support the growth of specific types of microorganisms. There are several types of microbiological media, classified based on their composition and purpose:
- Nutrient Agar: Nutrient agar is a general-purpose medium used to grow a wide variety of bacteria and fungi. It contains beef extract, peptone, agar, and water.
- Blood Agar: Blood agar is a differential medium used to differentiate bacteria based on their ability to hemolyze (break down) red blood cells. It contains nutrient agar supplemented with sheep or horse blood.
- MacConkey Agar: MacConkey agar is a selective and differential medium used to isolate and differentiate Gram-negative bacteria, particularly enteric bacteria. It contains bile salts and crystal violet to inhibit the growth of Gram-positive bacteria, and lactose and pH indicators to differentiate lactose fermenters from non-fermenters.
- Sabouraud Agar: Sabouraud agar is used to isolate fungi and yeasts from clinical specimens. It contains a low pH and high glucose concentration to inhibit bacterial growth and promote fungal growth.
- Eosin Methylene Blue (EMB) Agar: EMB agar is a selective and differential medium used to isolate and differentiate Gram-negative bacteria, particularly fecal coliforms. It contains eosin and methylene blue dyes to inhibit the growth of Gram-positive bacteria and differentiate lactose fermenters from non-fermenters.
- Mannitol Salt Agar: Mannitol salt agar is a selective and differential medium used to isolate and differentiate staphylococci, particularly Staphylococcus aureus, from other bacteria. It contains high salt concentration to select for staphylococci and mannitol and pH indicators to differentiate mannitol fermenters from non-fermenters.
- Thioglycollate Broth: Thioglycollate broth is a liquid medium used to culture anaerobic and microaerophilic bacteria. It contains thioglycollate to reduce oxygen tension and resazurin as an oxygen indicator.
These are just a few examples of the many types of microbiological media used in laboratory settings. The choice of medium depends on the specific requirements of the microorganisms being cultured and the goals of the experiment.